Author: Denis Noble
Affiliation:
CBE FRS, Balliol College, OX1 2BJ, and Department of Physiology,
Anatomy and Genetics, Parks Road, Oxford OX1 3PT, UK. E-mail:
[email protected]; Tel. +44-1865 272528; Fax +44-1865 272554.
http://musicoflife.co.uk/Title: (title to come)
Authors: Valdur Saks and Claire Monge
Affiliation:
INSERM U884, Laboratoire de la Bioénergétique et Appliquée, Université
Joseph Fourier, 2280 Rue de la Piscine, BP 53, Grenoble Cedex 9,
France. E-mail :
[email protected]; Tel. +33-476635627; Fax : +33-476514218.
Type: Review
Title: Philosophical and Historical Basis of Systems Biology Approaches: from Hegel to Noble. Application for Bioenergetic Reasearch
Abstract:
In our days, we live in the time of change of the paradigm of
biological sciences. Reductionism that used to be the philosophical
basis of biochemistry and molecular biology during last six decades
when everything, from genes to proteins and organelles - were studied
in their isolated state is leaving its place to Systems Biology that
favours the study of integrated systems at all levels: cellular, organ,
organism, and population. Reductionism was justified in the initial
stages of biological research giving a wealth of information on system
components. It is timely topic to put them together and analyze them in
interaction, to understand the principles of functioning of the whole.
However, in historical perspective first systems biology approach was
applied already by Claude Bernard about 150 years ago (see ref. 1 for
review). These developments follow very precisely the dialectical
principles of development from thesis to antithesis to synthesis
discovered by Hegel (Scheme 1).
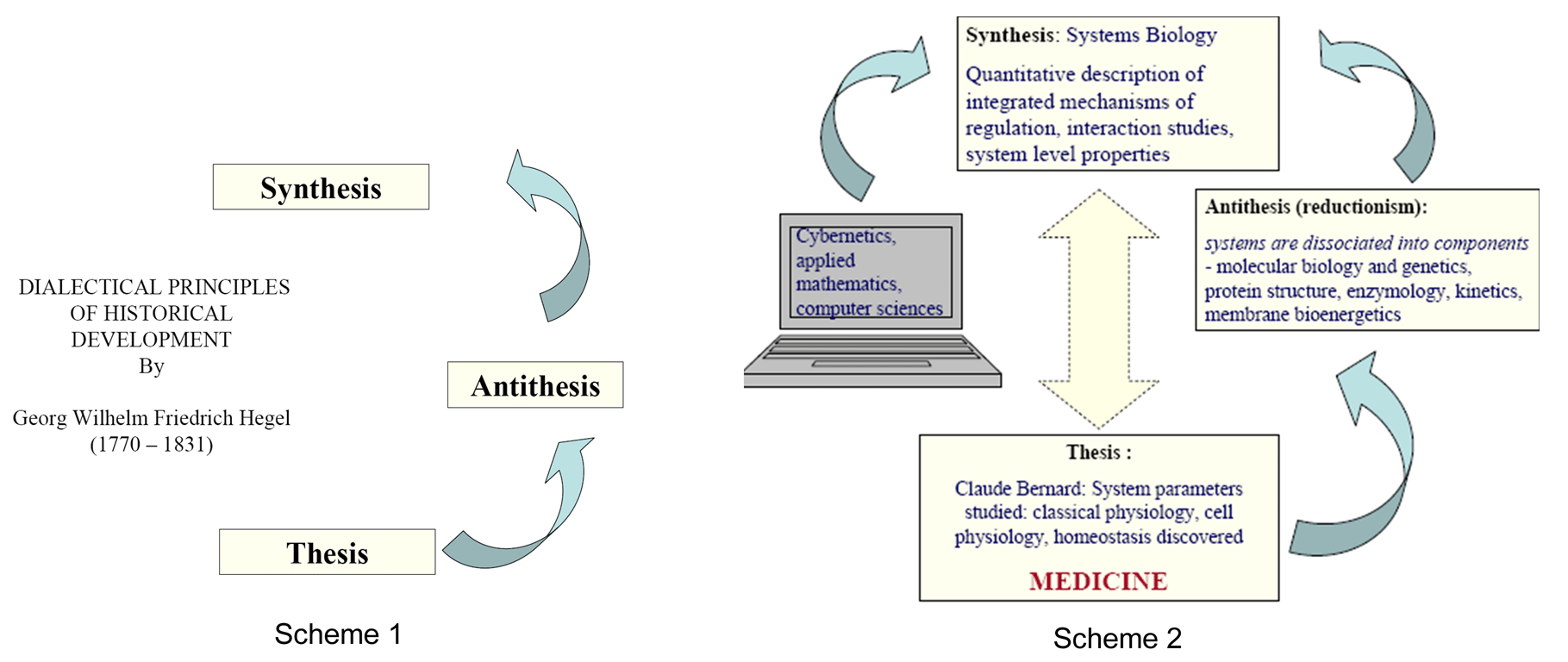
Dialectical
principles of Hegel can be perfectly and very logically applied for
description and analysis of the development of biological sciences
(Scheme 2). The aim of Systems Biology is the higher – level analysis
of complex biological systems (see ref. 2 and 3 for review) by using
the wealth of information obtained in studies of isolated components,
applying the methodological approaches of cybernetics, applied
mathematics, network analysis, nonequilibrium thermodynamics of open
systems (see ref. 4 for review) etc.
The Systems Biology
opens new perspectives for studies of the integrated processes of energy
metabolism in different cells (3). These integrated systems acquire new, system
- level properties due to interaction of
cellular components, such as metabolic compartmentation, channeling and
functional coupling mechanisms, which
are central for regulation of the energy fluxes. State of art of these studies
in the new area of Molecular System Bioenergetics is analyzed.
References:
1. Noble D. 2008. Claude Bernard, the first systems biologist, and the future of physiology. Exp. Physiol. 93.1: 16 - 26
2. Kitano H. 2002. Systems Biology: A brief overview. Science, 295: 1662-1664.
3. Noble D. 2006. The music of life. Biology beyond the genome; Oxford University Press: Oxford, UK.
4. Prigogine I., Strengers I (1986) La Nouvelle Alliance. Les Editions Gallimard, Paris.
Author: A. Kuznetsov
Affiliation: D. Swarovski
Research Laboratory, Department of Transplant Surgery, Innsbruck
Medical University (IMU), Innrain 66, A- 6020 Innsbruck, Austria. E-mail:
[email protected]; Tel. +43-512504-27811; Fax: +43-512504-24625
Type: Review
Title: Heterogeneity of Mitochondria and Mitochondrial Function within Cells: Another Level of Mitochondrial Complexity
Abstract:
Beyond fundamental role in energy metabolism mitochondria perform a
great variety of other important cellular functions. However, the
interplay among these roles of mitochondria is still poorly understood.
Importantly, mitochondria localized in different regions of a cell may
display different morphology (small spheres, short rod-like shape,
spaghetti-like shape, long branched tubules, complex mitochondrial
network), dissimilar biochemical properties, or may differently
interact with other intracellular structures. Recent advances in live
imaging technique have discovered also functional heterogeneity of
mitochondria in respect to mitochondrial redox state, membrane
potential, respiratory activity, uncoupling proteins, mitochondrial ROS
and calcium. Distinct mitochondrial subsets may also have different
responses to substrates and inhibitors and may vary in their
sensitivity to pathology, resistance to apoptosis, oxidative stress,
etc, demonstrating also heterogeneous dynamic motility, combining
different types of mitochondrial motion (e.g. small oscillatory
movements in mitochondrial network, filament extension, retraction,
fast and frequent oscillating branching and long-distance translocation
of single mitochondrion or mitochondrial filament). All these
observations show a new level of mitochondrial complexity and strongly
suggest that intracellular position and/or specific surrounding of
mitochondria within the cell may define their functional features,
suggesting also that different mitochondrial subpopulations, clusters
or even single mitochondrion may carry out diverse processes in a cell.
An important and still unresolved question is how heterogeneity of
mitochondrial function and regional specializations of mitochondria are
genetically defined and to which extent this heterogeneity may be
dependent on environmental aspects.
In addition, mitochondrial
defects can be heterogeneously expressed in different mitochondrial
subpopulations, which may be differently involved in pathological
processes. They may have diverse sensitivities to injury or be
associated with dissimilar metabolic consequences. The analysis of
metabolic and functional diversity of mitochondria, thus, have
important implications for analysis of mitochondria related pathologies
like ischemia-reperfusion injury, oxidative stress, various
cytopathies, etc. The application of mitochondrial imaging opens a very
promising avenue for the development of new diagnostic approach for the
detection of regional mitochondrial defects. Analysis of heterogeneity
of mitochondria and mitochondrial function therefore represents a new
challenging area in the mitochondrial research, potentially leading to
the integration of mitochondrial bioenergetics and cell physiology with
various physiological and pathophysiological implications.
Authors: Aon Miguel and Sonia Cortassa
Affiliation:
Johns Hopkins Univ. School of Medicine, Institute of Molecular
Cardiobiology, 720 Rutland Ave. 1055 Ross Bldg., Baltimore, MD
21205, USA. E-mails:
[email protected],
[email protected]u; Tel. +1-(410) 955-2759; Fax: +1-(410) 955-7953
Type: Review
Title: Control and Regulation in Metabolic and Transport Networks of Integrated Cardiomyocycte Function
Authors: Dzeja Petras and André Terzic
Affiliation:
Division of Cardiovascular Diseases, Departments of Medicine, Molecular
Pharmacology and Expermental Therapeutics, Mayo Clinic College of
Medicine, Guggenheim 7, Rochester, MN, 55905 USA. E-mail :
[email protected],
[email protected]; Tel. +1-507 2660606
Type: Article
Title: (title to come)
Authors: Marko Vendelin and J. Engelbrecht
Affiliation: Laboratory of Systems Biology, Institute of Cybernetics, Akadeemia tee 21, 12618 Tallinn, Estonia.E-mail:
[email protected],
[email protected]; Tel. 372 620 4160; Fax: 372 620 4151
Type: Article
Title: (title to come)
Authors: Jacques Demongeot
Affiliation:
Laboratoire TIMC-IMAG UMR 5525 - Pavillon Taillefer, Faculté de
Médecine de Grenoble - 38700 La Tronche. Tel. 33 (0)4 56 52
01 08; Fax: 33 (0)4 56 52 00 44
E-mail:
[email protected]Title: Robustness in Regulatory Networks. A Generic Approach with Applications at Different Levels: Physiologic, Metabolic and Genetic
Abstract: The regulatory networks
are often studied on their dynamical side (existence of attractors, study of
their trajectory stability) but rarely on their robustness, that is their
ability to resist to external perturbations, then offering the same
spatio-temporal patterns independently of the loss of nodes or edges in their
interaction architecture, or of the change in their environmental boundary
conditions, or more of their mode of updating (e.g. co-expression versus
sequential expression in genetic networks). We will define precisely the generic
notion of critical node or edge in the interaction graph whose disappearance
can cause dramatic changes in the number and type of attractors (e.g. passage
from a bistable behavior to a unique periodic regime) or in the range of their
basins of stability. We will treat some examples: bulbar cardio-respiratory
physiologic regulation, cell cycle genetic control and feather morphogenesis
metabolic driving.